A Heart-healthy Laser
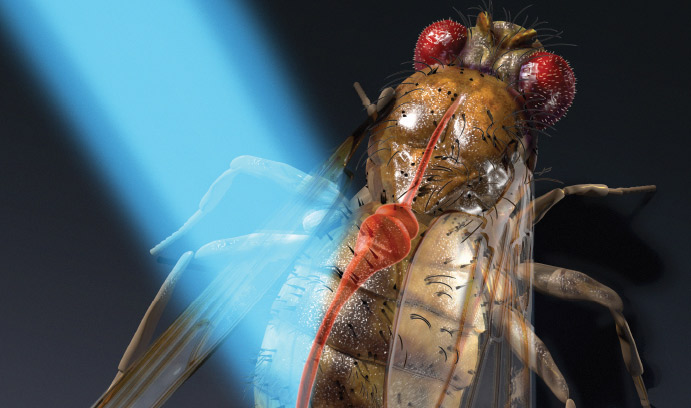
Chao Zhou's work proved that bursts of light could be used to manipulate the heartbeat of fruit flies. Human applications are possible as well.
As electrical systems go, the human heart is one of nature’s most durable. Every minute, your heart beats about 80 times; every hour, nearly 5,000 times. By the time you are 70, you will have recorded about 3 billion heartbeats.
Each of those heartbeats is a marvel of coordination between the heart’s four chambers—the right and left atria, and the right and left ventricles. As the atria fill with blood, an electrical signal is generated that causes both to contract and push blood into the ventricles. The ventricles then contract and push blood through the pulmonary and aortic valves and out to the rest of your body. The ventricles then relax, the atria fill again with blood, and the cycle repeats.
If injury or disease disrupts your heart’s electrical system, you can be fitted with a battery-powered pacemaker that signals the cardiac tissue, helping your heart regain its regular rhythm. Electric pacemakers are used today by an estimated 3 million people around the world.
Pacemakers have helped scientists learn more about the heart’s physiology and its disorders, says Chao Zhou, but they have their limits. They must be surgically implanted. They are not specific to heart tissues and can cause unwanted contractions in other areas of the chest. They produce inhomogeneous areas of depolarization and can also generate toxic gases, alter pH levels and cause tissue damage.
Zhou, assistant professor of electrical and computer engineering, and his colleagues have taken the first step toward developing a laser pacemaker that could one day stimulate the human heart noninvasively with light signals.
The researchers have built a microscope that paces the tiny heart of the common fruit fly without touching it, while simultaneously controlling its heart function, monitoring its performance and taking high-resolution images of it at the microscale.
The system uses two optical technologies—optical coherence tomography (OCT) and optogenetics—to generate pulsed blue light signals that pace the fruit fly’s heart during the three stages of its life: larva, pupa and adult.
The group reported its results recently in Science Advances, a journal published by Science magazine. Their article, titled “Optogenetic pacing in Drosophila melanogaster,” was written by Aneesh Alex, a former postdoctoral research scientist at Lehigh, and co-authored by Zhou and by Airong Li and Rudolph E. Tanzi, both of the Genetics and Aging Research Unit of Harvard Medical School’s Department of Neurology. The project has been funded by the National Institutes of Health (NIH).
Optogenetics uses light to control and study the activities of living cells that have been genetically modified with a light-sensitive protein. OCT combines light waves, usually from the near-infrared portion of the electromagnetic spectrum, with interferometry to capture microscale images from deep within biological tissue and other media that scatter optical signals.
Optogenetics was invented by researchers at Stanford University a decade ago to study nerve cells. In 2010, says Zhou, two other groups published articles about the use of optogenetics to pace the heart of the zebrafish. At that time, Zhou, a postdoctoral researcher at the Massachusetts Institute of Technology, was using OCT to study fruit flies in collaboration with researchers from Harvard Medical School.
“NIH had awarded me a grant to improve OCT technology to enable the molecular imaging of cell features,” says Zhou. “I came up with a new idea, which was to use optogenetics to stimulate heart pacing and OCT to monitor heart function.
“We found that we could shine a light to control the heart rhythm and then use OCT to confirm that the heart was beating and to see how it was beating. We used OCT to see the fly’s heartbeat in real time.”
The fruit fly, known scientifically as Drosophila melanogaster, offers several advantages for optical study, says Zhou. First, biologists have obtained the complete genome sequence for the fruit fly and developed many ways to modify the genome. Second, despite differences in scale and complexity between humans and fruit flies, Zhou says, the genomes of the two members of the animal kingdom are surprisingly similar.
“For human beings and fruit flies,” says Zhou, “70 to 80 percent of the genome is the same. You can easily test a human gene type in the fruit fly and then in a mouse.”
The heart of a typical adult fruit fly lies about 200 microns below the fly’s outer tissue surface. It varies in size between 10 and 20 microns when contracting and about 100 microns when dilating. And the heart beats 300 to 400 times per minute.
“It is possible to observe the heartbeat of an intact fly with near-infrared OCT technology,” says Zhou. “And because the fruit fly heart is so small, we need a very sophisticated imaging technique with a high resolution that works very quickly. Otherwise, the image we get is very blurry.”
To begin their experiments, Zhou and his group bred a genetically altered strain of fruit flies by inserting a light-sensitive protein (taken from algae) into their cardiac cells. Then they anesthetized a fly, taped its wings to a glass microscope slide and shined a low-power, 16-milliwatt blue laser at its heart. When the researchers changed the frequency of the laser, they observed a corresponding change in the heart rate of the fly. When the laser pulsed 10 times per second, for example, the heart rate accelerated to 10 beats per second, in perfect synchronicity with the laser.
To monitor the fruit fly’s response to the laser, Zhou and his group developed an optical coherence microscopy (OCM) system that enables nondestructive imaging in real time at microscale resolution. Using OCM, the researchers were able to monitor and analyze the structure and function of the fly heart at the larva, pupa and adult stages.
“OCT is like ultrasound, which sends high-frequency sound waves into tissue and then measures the reflection,” says Zhou.
“OCT is similar. It uses near-infrared light instead of sound. The light has a shorter wavelength than the sound waves, which gives us much finer resolution, at the microscale. As the light is reflected from different depths of tissue, the delay in the reflecting of the light signal tells you how deep the signal has penetrated into tissue. From this, we can generate a cross section of a sample from beneath the surface of the tissue.”
The use of optical coherence microscopy, the researchers wrote in Science Advances, allowed the group to “quantitatively determine cardiac physiological parameters, such as refractory period [recovery time] and contraction time, of the Drosophila heart at different stages of its life cycle.”
After the group concluded its experiment, the fly continued its normal development.
“One of the key advantages of our system is that it is completely noninvasive,” says Zhou. “We can do experiments over and over again on the same specimen.
“If you sacrifice animals during experiments, you obviously cannot use them again. This makes it difficult, if not impossible, to study the developmental stages of the same animal. With noninvasive optical stimulation and imaging, however, you can see how the same fly heart grows and develops.”
During the course of their three-year study of the fruit fly, Zhou and his collaborators have observed that the fly’s heart slows down in the early pupal stage, stops beating for about a day, and then resumes beating and restores its normal heart rate of 300 to 400 beats per minute in the late pupal and adult stages.
“We were the first group to observe this dynamic change in the fly heart rate,” says Zhou. “Nothing in the literature showed this. It is difficult to detect it without the noninvasive imaging tools we have.”
Zhou’s group has also used optical coherence microscopy to study the influence of the circadian rhythm gene on the fruit fly heart. Circadian rhythms refer to oscillations in an organism’s physical, mental and behavioral processes, including levels of hormones expressed. These rhythms result from environmental cues. They often follow a 24-hour cycle, and they vary from day to night.
In a paper published in September 2015 by PLOS ONE (the Public Library of Science), Zhou and his collaborators from Harvard Medical School, joined by researchers from Zhengzhou University in China, reported that the circadian clock gene plays a major role in the development of the structure and function of the fruit fly heart.
Their study, the researchers said, was one of the first to be conducted in a whole animal in vivo that explored the role that circadian clock genes play in heart development and function.
In the PLOS ONE article, Zhou’s group reported that expression levels of one of the fruit fly’s circadian clock genes, cryptochrome (dCry), increase significantly during the fly’s pupal and adult stages. Reductions in those levels, they wrote, “resulted in a slower heart rate, a reduced cardiac activity period, a smaller heart chamber size, pupal lethality and disrupted posterior segmentation.
“Collectively, our studies provided novel evidence that the circadian clock gene … plays an essential role in heart morphogenesis and function.”
Zhou, who recently received a second grant from the NIH’s National Institute of Biomedical Imaging and Bioengineering to explore different pacing strategies using OCM, says it will take many years before optical pacemakers can be used in humans.
“Our initial goal was not to develop optogenetic pacing for humans,” he says. “That day is very far off. Instead, we wanted to do this in small animals in order to verify and refine the effectiveness of using near-infrared light signals to stimulate and image heart muscle cells. Also, we wanted to find out how different genes affect heart development. To learn this, we first needed to develop a good research tool.
“With bigger animals come challenges. How do you insert light-sensitive proteins? How do you do near-infrared simulation? An optical fiber or LED would need to be implanted, which is invasive. Also, near-infrared light waves will scatter, but without being completely lost. You would have to guide this light to the region where you want it in order to do optical pacing.”
Improving the ability to do noninvasive pacing with optical signals, however, can also help advance the development of novel medical treatments, says Zhou.
“For example, if a person is born with a congenital defect in his heart, can we shine a light on his heart, when he is in his early developmental stages, to help correct the defect? Can we use therapeutic tools to correct human diseases?
“We don’t know the answer to these questions yet. We’re still at the hypothesis stage, but the technology now exists to make it possible to think about them.”
Shedding New Light on Biopsies
Physicians often take biopsies to make clinical diagnoses. Tissue samples removed from the patient are placed in wax, cut into very thin slices, stained and examined under a microscope. This process is labor-intensive and takes time, leaving worried patients waiting for days or sometimes weeks for results. During surgery, removed tissue samples are sent for similar histological evaluations. Some patients must return for a second surgery if doctors discover that a portion of a tumor remains after the original surgery.
“It’s a clinical practice, but it’s not ideal,” says Zhou.
He and his team think there’s another way: the optical biopsy. Optical coherence tomography (OCT) and optical coherence microscopy (OCM) have the potential to change the face of cancer detection and evaluation. Conceptually similar to ultrasound but using near-infrared light instead of sound, optical biopsies can reduce or even eliminate the need for tissue excision and processing by providing in situ 3-D structural and functional images of tissue microstructure with a cellular resolution close to that of histology. With OCT and OCM, results can be obtained within minutes.
Zhou and his colleagues conducted a study in which three pathologists blindly read their images of human kidney samples. They then compared the diagnoses, which were remarkably accurate: over 80 to 90 percent.
“We send the light in, detect the reflected light, and we figure out where the reflected light is coming from. That gives us depth information with micron-scale accuracy. ... Once you know from a single point what the reflectivity of different depths [of tissue] is, then you can move your light beam along the tissue surface and get a cross-sectional image of the tissue. Then you move the beam over a square and you get an entire 3-D picture of the sample,” explains Zhou.
Right now, optical biopsies face practical limitations. Depth, for example, is a challenge. So far, OCT and OCM work only with some superficial tumors such as skin cancers. In cases of breast cancer, an imaging probe might aid during surgery to identify areas of potential concern and possibly help reduce the need for a second surgery. For now, Zhou is working on how they might improve the surgical procedures using this technique.
“We’re pretty optimistic that with further development, we can make this real-time optical biopsy possible, and that can hopefully change the clinical practice,” says Zhou.
Chao Zhou’s research interests include biomedical optics, optical coherence tomography (OCT), optical coherence microscopy (OCM), diffuse optical spectroscopy/imaging and laser speckle imaging. He received his Ph.D. from the University of Pennsylvania and served as a postdoctoral associate at the Massachusetts Institute of Technology.
Story by Kurt Pfitzer
Illustration by Nicolle R. Fuller
Posted on: