A New Vision for Glass
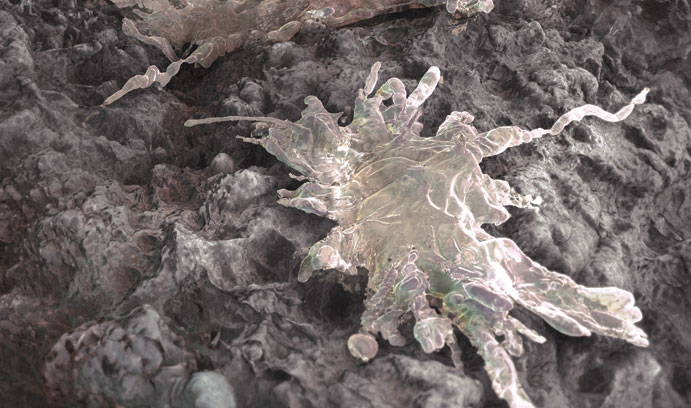
Himanshu Jain and his colleagues have placed glass at the forefront of biomedicine. Here, bone-forming cells (rendered in white) attach to the biologically receptive TAMP surface to send out extensions to explore the scaffold microenvironment.
Himanshu Jain has a knack for engaging in conversations with just the right people at just the right time. In 2004, a casual chat with the person sitting next to him at a banquet in Cairo, Egypt, ended up guiding much of his research over the next decade. The result—thanks in part to additional fortuitous hobnobbing later—has been groundbreaking advances in a significant area of biomedical materials research.
Jain, the T.L. Diamond Distinguished Chair in Engineering and Applied Science and professor of materials science and engineering, had his momentous conversation at a workshop with colleagues from the United States and Africa. Seated next to him was Mona Marei, who headed the tissue-engineering lab at Alexandria University's Faculty of Dentistry. "We started chatting, and when I said I worked on glass, she said, 'You guys are no good,'" Jain says. "I was kind of taken aback. I said, 'Tell me why you think that way.'"
Marei had been using implanted biocompatible glass to repair deteriorated or damaged teeth and bone but wasn't happy with her results. After the Cairo workshop, Jain visited Marei's lab to learn more. "I had no serious understanding of the human body, let alone bone," he says. "But I found the whole idea of tissue engineering intellectually very exciting."
Jain had decades of experience developing sophisticated forms of glass for biomedical sensors, communications and microelectronics. In 2004—with funding from the National Science Foundation—he helped establish the International Materials Institute for New Functionality in Glass at Lehigh. The Cairo workshop was an introductory event for NSF International Materials Institute participants.
NSF was seeking to promote international research collaborations. Jain wanted to revitalize glass research and education, which was declining nationally due to glass's energy-intensive costs and to global competition. "I didn't want to see glass in this country go the way of steel," Jain says. "We needed more value-added glass products that require research and innovation."
RICH IN PORES
For hundreds of years, scientists had sought to make glass that lasts. "Like with windows," Jain says. "They last for centuries." Marei's challenge—and a key to future medical applications—was to create a biomedically active glass that goes away after it has served its purpose.
That purpose was to provide a "scaffold" that would help the body regenerate its own bone. It differs from conventional body part replacements, especially of hard tissues, which usually rely on long- lasting synthetic implants. "The body doesn't regenerate most organs by itself," Jain says. "But if you provide the right kind of microenvironment, it can."
Researchers had already established that glass can be made biocompatible, meaning the body will accept it and not reject it as a foreign substance. Jain's contributions have been to learn to control glass's biodegradation and to foster its ability to regenerate bone.
For glass to promote healthy bone growth and then disappear required it to do things it hadn't done before. "We started with the simple sense of how substances dissolve in liquid," Jain says. "If you have a solid crystal of sugar in coffee, it takes a long time to dissolve. If you have fine-grain sugar, it dissolves quickly."
The difference is that a grain of sugar has a proportionally much larger surface—the most reactive portion of a material—than does a large crystal. So Jain's group sought to make bioactive glass porous, with interconnected macropores measuring 100 to 200 microns (millionths of a meter) in diameter. The macropores would also allow space inside the glass for cells, nutrients and waste to move, and for blood vessels to form—key factors in regenerating bone, which is naturally porous.
But Jain and his colleagues envisioned making bioactive glass porous on not just one, but two levels—and the levels seemingly didn't go together. "We knew how to make large pores," Jain says. "The challenge was to introduce nanopores at the same time." Nanopores measuring about 10 nanometers—10,000 times smaller than macropores—would vastly expand the material's surface area and potentially offer a means of fine-tuning the glass's biodegradation.
The problem: "Small pores are unstable in the presence of big ones," Jain says. "We had to come up with ways to make sure both can coexist and remain in the glass when it's made." The key lay in phase separation, a condition in which—as with oil and vinegar in a salad dressing—two distinct materials with different properties exist at the same time in one substance. "Think of pores as another material," Jain says. "Our goal was to have a composite of glass and pores."
A team of Lehigh and international colleagues, including researchers from the Instituto Superior Técnico, in Lisbon, Portugal, and Alexandria University in Egypt, developed or refined two glass processes that successfully resulted in dual porosity—that is, pores at both macro and nano scales. One method is not far removed from traditional ways of making glass. Called melt quench, it entails mixing and melting oxides of calcium, phosphorus and silicon; casting as glass; heat-treating for desired phase separation; and then selectively leaching out appropriate phases to obtain multiporous glass. The other process, sol-gel, uses relatively low temperatures and forms a phase-separated gel which is then heat-treated to drive off the liquid.
Each technique presents a different balance of advantages and disadvantages. Dually porous, bioactive glass made with the melt quench method is stronger but less porous and somewhat inhomogeneous. Sol-gel glass is more readily fine-tuned at the nano scale, but the process is more time-consuming and expensive.
"This is all just the materials science," Jain says. "Getting it into patients is a very different matter."
THE 'BIO' IN BIOMATERIAL
Jain now realized the project needed a biologist. (To gain background, he himself had once taken a Lehigh cell biology course in which his daughter was a student. "She said, 'No way!' and I said, 'I promise I'll sit on the other end of the classroom,'" Jain says with a laugh.)
As with his initial conversation with Marei, Jain had another providential travel encounter. This time, he was flying to Japan for an international symposium on new materials science. Seated next to him was Matthias Falk, associate professor of biological sciences at Lehigh, who was traveling to Japan for an international microscopy congress. They looked familiar to each other, quickly discovered they were both from Lehigh and started to discuss their work.
"One aspect Himanshu explained was that they were having trouble detecting cells on the glass, which is not transparent," Falk says. "Neither light microscopy nor reflection microscopy worked very well. I said I thought we had a solution."
Falk's research on gap junctions, through which cells communicate with each other, often uses fluorescent microscopy, in which fluorescent dyes make cells stand out. Falk seeded samples of Jain's multiporous glass with human osteosarcoma cancer cells and mouse bone cells and found that using fluorescent microscopy vastly improved the visibility of cells on the material compared to the methods Jain had been using.
Overall, Falk found Jain's glass project exciting. "If an engineer can design a material that can go into the body, have cells bind to it, regenerate natural material and then degrade—that's amazing," he says.
Falk began investigating how well cells bind to multiporous bioactive glass. The results were better than expected. "Cells attach strongly to the material," Falk says. "They really seem to like it."
Bone cells on a multiporous bioactive glass have now been shown to grow deep into the scaffold and secrete proteins that help build new natural bone. What's more, cells for unknown reasons seem to prefer material with smaller nanopores to material with larger nanopores or no pores at all. That suggests engineers can modulate cell function or tissue regeneration rates by changing the nanoporosity of the scaffolding. "How do cells know whether a pore is five nanometers or 50?" Jain says. "We're trying to establish a fundamental understanding of how nanoscale morphology is important to cells."
Pore size also affects how quickly the scaffolding material breaks down. Producing what's known as a tailored amorphous multiporous (TAMP) structure potentially allows many different applications in dissimilar parts of the body where the best results may depend on varying properties in the material.
Jain, Falk and their colleagues are now exploring additional biomedical applications for multiporous bioactive glass. The material may be useful for in vitro fertilization, potentially improving the development of embryos before they're implanted. Doctors at Lehigh Valley Health Network have shown interest in the material's potential to help heal burns and chronic wounds. Collaborators at the Central Glass and Ceramic Research Institute in Kolkata, India, have adapted the technology to make an injectable paste to repair osteoporotic vertebral compression fractures in elderly patients. The material could even be used as a vehicle for delivering continuously released medication.
Meanwhile, back in Egypt, Mona Marei has tested the material in goat and dog mandibles and under the skin in rabbits. She's now begun to test it further in ways she had dreamed back in 2004—rebuilding bone in humans receiving tooth implants or undergoing facial reconstruction. Preliminary results of a clinical trial on children started in her lab last year have been encouraging.
"She's pretty convinced this is promising and that multiporous bioactive glass is the right material for a number of applications in dentistry," Jain says. "Our work has come back to where it started, and this for me is hugely satisfying."
Real-world Impact
When a tooth is extracted, the surrounding alveolar bone that supports the teeth begins to degenerate. Alveolar bone support is essential to the successful placement of dental implants, and in some cases implants cannot be placed without a bone-grafting procedure.
Mona Marei’s work with nano-macro porous bioactive glass in orthodontics has shown great promise. Hoping to utilize Lehigh bioactive glass to promote healing of the extraction socket while preserving the height and width of the bone, she and her team in the Faculty of Dentistry at Alexandria University have initiated a clinical trial of patients aged 9-16 years with malocclusion and good oral hygiene. Marei and her colleagues extracted the maxillary and mandibular premolar teeth of each patient. They then used sterile saline to moisten TAMP powder to a desired consistency before inserting it into one empty socket and securely suturing it. They left the contra lateral extraction socket unfilled as a control and followed up with patients clinically and radiographically after one week, two weeks, and one, two, three and six months post-surgery.
The study’s preliminary findings show promising results of bone regeneration and are consistent with previous data from their animal studies. Of particular note are the differences in bone regeneration patterns between the treated and untreated extraction sockets and the “remarkable” growth of new blood vessels from existing blood vessels in the bioactive TAMP powder-filled socket.
“From my perspective, it is particularly exciting, in fact amazing, that our TAMP composition stimulates the regeneration of both hard and soft tissues as observed by Dr. Marei and her team,” says Jain.
Himanshu Jain is the director of the International Materials Institute for New Functionality in Glass. His research interests include introducing new functionality in glass through fundamentals; nano-macro porous glass for tissue engineering; light-induced phenomena in glass; and glasses for IR biosensors, photo- and nano-lithography, and photonics. He received his D.Eng.Sc. from Columbia University.
Matthias Falk received his Ph.D. from the University of Heidelberg, Germany. He was trained as a cell biologist and is interested in the processes related to protein biosynthesis, intracellular trafficking, the assembly of multi-protein complexes and how cells in multi-cellular organisms communicate with each other. Before coming to Lehigh, Falk worked at The Scripps Research Institute (TSRI) in La Jolla, California.
Story by Rich Laliberte
Illustration by Markos Kay
Posted on: