The cooperative effects of tension and elasticity
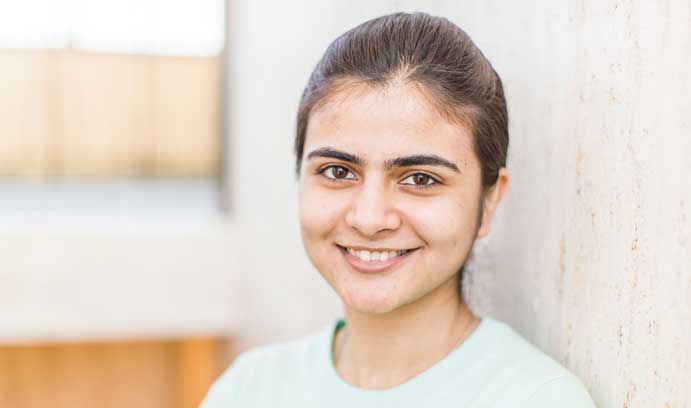
Aditi Chakrabarti found that a nonmagnetic attraction causes particles and particle clusters suspended in a gel to form spontaneous three-dimensional assemblies.
It was a straightforward experiment: drop tiny solid spheres of metal and ceramic into a gel material and see what they would do.
Aditi Chakrabarti, a Ph.D. candidate in chemical engineering, wasn’t certain how the particles would behave, but she was hoping to see something no one had seen before.
What she saw surpassed her expectations.
Looking through a clear, glass container, Chakrabarti watched the spheres sink to a particular depth where they became stagnant in the polyacrylamide gel, which is a soft elastic solid.
Her experiments showed that the particles, which measured 2 to 5 millimeters in diameter, not only sank into the gel but began to interact with each other, eventually forming close-packed structures, in a process resembling crystallization.
After doing further experiments with combinations of copper, steel, glass and ceramic spheres, Chakrabarti discovered that the forces governing the movements of the particles were the result of the cooperative effects of surface tension, elasticity and gravity.
This particular phenomenon—an “emergent phenomenon that leads to an effective force,” she said—had never been seen before, nor had it been predicted.
“A plethora of interesting phenomena”
Chakrabarti wrote an article about her discovery that was published last December in the prestigious chemical engineering journal Langmuir: The ACS (American Chemical Society) Journal of Surfaces and Colloids. She also contributed the cover art for the issue, which depicted the interaction of the particles in the gel.
Her paper, coauthored with her adviser, Manoj Chaudhury, the Franklin J. Howes Distinguished Professor of Chemical Engineering, was titled “Surface Folding-Induced Attraction and Motion of Particles in a Soft Elastic Gel: Cooperative Effects of Surface Tension, Elasticity and Gravity.”
In the paper, Chakrabarti, wrote that her theory, which is based purely on experimental observations, holds that the cooperative effects of surface tension and elasticity gave rise to a “plethora of other interesting phenomena.”
Scientists have long known that objects can interact because of the mechanical distortion of the medium that surrounds them as well as physical forces like elasticity and gravity. The movements of proteins and other cell components, for example, are governed in part by elastic forces within the cell environment.
In Chakrabarti’s experiments, a particle inside the polyacrylamide gel appears to be moving through a thick liquid. However, it is not displacing the gel the way it would displace water. Instead, the tiny sphere experiences not only gravity but also elastic forces as the soft solid of the gel is stretched around and over it.
After watching a single, 2-millimeter-sized copper particle sink to a certain level and reach a state of equilibrium inside the gel, Chakrabarti dropped a second particle a few millimeters away from the first and watched as it sank to the same depth and stopped. The second particle then started moving towards the first sphere until it made contact and created a dimer, a structure of two identical units.
When a third particle was released, it moved toward the dimer and formed a triangular structure. A fourth released particle formed a tetrahedron. As Chakrabarti continued adding copper spheres, they formed a large cluster on one side of the gel screen.
Likewise, structures of two or more particles were also attracted to each other. They moved closer together and eventually made contact, forming larger clusters.
Curious to see how multiple clusters would interact, Chakrabarti formed a second cluster on the other side of the gel. The result was surprising.
“When these two clusters, still suspended in the gel, were big enough, they started coming together until they made contact and reorganized, forming a close packed structure. Not only did they make contact with each other, but they became kind of clenched together, almost like crystallization,” she said, interlocking and tightening her fists. The process made her think of a kind of spontaneous 3D assembly.
“People have done lots of 2D assembly in liquids. But I’m not sure 3D assembly in this kind of a soft gel has been done. It’s new,” she said.
A non-magnetic attraction
Chakrabarti made videos of her experiments using a CCD (charge-coupled device) camera equipped with a microscope. The particles look like two magnets attracted to each other, but no magnetic force is involved. Before Chakrabarti drops a sphere onto the surface of the gel, the surface is straight. Each time she drops a sphere, it plunges into the gel, deforming the gel surface. The gel folds and closes around the top of the sphere due to hoop stress and surface tension, leaving a thin channel from the sphere to the surface. It’s as if the gel stitches itself up again around the sphere, Chakrabarti says. The sphere’s effective weight is balanced by the deformed elastic field inside the gel.
When she drops another sphere some distance away, the same thing happens. As the gel is being deformed in two places, tensile stress fields form in the gel parallel to the surface. Nothing counterbalances this stress field, so the gel between the two spheres is squeezed out of the way, allowing the two spheres to approach each other and finally touch. The same interaction occurs between two multi-sphere structures in the gel.
Chakrabarti found that the attraction is due to the difference between the potential energy released as the sphere descends and a subsequent increase in the elastic energy. The attraction between two spheres depends on the relationship between the depth to which they are submerged and the separation between them. She used an image analysis software called ImageJ to measure the change in depth of the particles and the distance between two particles as they move towards each other.
The experiments, said Chaudhury, represent “the discovery of an effective force that hasn’t been seen before, by combining all of these known components: gravity, capillary action and elasticity. This work illustrates that by the interception of various forces—elasticity, capillarity and gravity—it is possible to tune in a new type of interaction that would otherwise not exist without the cooperative e?ects of its parts.”
Chakrabarti is developing a model for particle behavior at the mesoscale—at which particles are smaller than the spheres she used, but larger than atoms or molecules. Such a model is the scientific starting point for further research that could one day be used for 3D crystallization of structures at the mesoscale.
A concrete application is still probably years away, Chaudhury says. But Chakrabarti has made a new scientific discovery, one that will fuel future research by other scientists and engineers. The two researchers hope to explore possible applications of Chakrabarti’s phenomenon in the fields of soft actuators and self-assembly processes. Their goal is to accomplish this by combining the properties of a gel with those of liquid crystal, whose elastic fields are self-induced by the interactions of the particles in the surrounding medium.
So far, no rigorous theory has been developed to account for the phenomenon that requires solutions of nonlinear field equations. However, Chakrabarti and Chaudhury have been able to develop a model at a scaling level that captures the main physics underlying the phenomenon.
Chakrabarti anticipates completing her Ph.D. in 2017 and pursuing an academic career. She also published a Langmuir paper with Chaudhury in May 2013 that describes their analysis of elastocapillarity in adhesion-induced instability in thin films and the surface tension of the soft elastic hydrogel that she used in her later experiments.
Since the second Langmuir paper was published, Chakrabarti and Chaudhury have continued experimenting with particles in the gel. They have also submitted for publication an article that presents a more detailed model to account for particle interaction that occurs on the gel’s surface.
Photos by Christa Neu
Aditi Chakrabarti, a Ph.D. candidate in chemical engineering, wasn’t certain how the particles would behave, but she was hoping to see something no one had seen before.
What she saw surpassed her expectations.
Looking through a clear, glass container, Chakrabarti watched the spheres sink to a particular depth where they became stagnant in the polyacrylamide gel, which is a soft elastic solid.
Her experiments showed that the particles, which measured 2 to 5 millimeters in diameter, not only sank into the gel but began to interact with each other, eventually forming close-packed structures, in a process resembling crystallization.
After doing further experiments with combinations of copper, steel, glass and ceramic spheres, Chakrabarti discovered that the forces governing the movements of the particles were the result of the cooperative effects of surface tension, elasticity and gravity.
This particular phenomenon—an “emergent phenomenon that leads to an effective force,” she said—had never been seen before, nor had it been predicted.
“A plethora of interesting phenomena”
Chakrabarti wrote an article about her discovery that was published last December in the prestigious chemical engineering journal Langmuir: The ACS (American Chemical Society) Journal of Surfaces and Colloids. She also contributed the cover art for the issue, which depicted the interaction of the particles in the gel.
Her paper, coauthored with her adviser, Manoj Chaudhury, the Franklin J. Howes Distinguished Professor of Chemical Engineering, was titled “Surface Folding-Induced Attraction and Motion of Particles in a Soft Elastic Gel: Cooperative Effects of Surface Tension, Elasticity and Gravity.”
In the paper, Chakrabarti, wrote that her theory, which is based purely on experimental observations, holds that the cooperative effects of surface tension and elasticity gave rise to a “plethora of other interesting phenomena.”
Scientists have long known that objects can interact because of the mechanical distortion of the medium that surrounds them as well as physical forces like elasticity and gravity. The movements of proteins and other cell components, for example, are governed in part by elastic forces within the cell environment.
In Chakrabarti’s experiments, a particle inside the polyacrylamide gel appears to be moving through a thick liquid. However, it is not displacing the gel the way it would displace water. Instead, the tiny sphere experiences not only gravity but also elastic forces as the soft solid of the gel is stretched around and over it.
After watching a single, 2-millimeter-sized copper particle sink to a certain level and reach a state of equilibrium inside the gel, Chakrabarti dropped a second particle a few millimeters away from the first and watched as it sank to the same depth and stopped. The second particle then started moving towards the first sphere until it made contact and created a dimer, a structure of two identical units.
When a third particle was released, it moved toward the dimer and formed a triangular structure. A fourth released particle formed a tetrahedron. As Chakrabarti continued adding copper spheres, they formed a large cluster on one side of the gel screen.
Likewise, structures of two or more particles were also attracted to each other. They moved closer together and eventually made contact, forming larger clusters.
Curious to see how multiple clusters would interact, Chakrabarti formed a second cluster on the other side of the gel. The result was surprising.
“When these two clusters, still suspended in the gel, were big enough, they started coming together until they made contact and reorganized, forming a close packed structure. Not only did they make contact with each other, but they became kind of clenched together, almost like crystallization,” she said, interlocking and tightening her fists. The process made her think of a kind of spontaneous 3D assembly.
“People have done lots of 2D assembly in liquids. But I’m not sure 3D assembly in this kind of a soft gel has been done. It’s new,” she said.
A non-magnetic attraction
Chakrabarti made videos of her experiments using a CCD (charge-coupled device) camera equipped with a microscope. The particles look like two magnets attracted to each other, but no magnetic force is involved. Before Chakrabarti drops a sphere onto the surface of the gel, the surface is straight. Each time she drops a sphere, it plunges into the gel, deforming the gel surface. The gel folds and closes around the top of the sphere due to hoop stress and surface tension, leaving a thin channel from the sphere to the surface. It’s as if the gel stitches itself up again around the sphere, Chakrabarti says. The sphere’s effective weight is balanced by the deformed elastic field inside the gel.
When she drops another sphere some distance away, the same thing happens. As the gel is being deformed in two places, tensile stress fields form in the gel parallel to the surface. Nothing counterbalances this stress field, so the gel between the two spheres is squeezed out of the way, allowing the two spheres to approach each other and finally touch. The same interaction occurs between two multi-sphere structures in the gel.
Chakrabarti found that the attraction is due to the difference between the potential energy released as the sphere descends and a subsequent increase in the elastic energy. The attraction between two spheres depends on the relationship between the depth to which they are submerged and the separation between them. She used an image analysis software called ImageJ to measure the change in depth of the particles and the distance between two particles as they move towards each other.
The experiments, said Chaudhury, represent “the discovery of an effective force that hasn’t been seen before, by combining all of these known components: gravity, capillary action and elasticity. This work illustrates that by the interception of various forces—elasticity, capillarity and gravity—it is possible to tune in a new type of interaction that would otherwise not exist without the cooperative e?ects of its parts.”
Chakrabarti is developing a model for particle behavior at the mesoscale—at which particles are smaller than the spheres she used, but larger than atoms or molecules. Such a model is the scientific starting point for further research that could one day be used for 3D crystallization of structures at the mesoscale.
A concrete application is still probably years away, Chaudhury says. But Chakrabarti has made a new scientific discovery, one that will fuel future research by other scientists and engineers. The two researchers hope to explore possible applications of Chakrabarti’s phenomenon in the fields of soft actuators and self-assembly processes. Their goal is to accomplish this by combining the properties of a gel with those of liquid crystal, whose elastic fields are self-induced by the interactions of the particles in the surrounding medium.
So far, no rigorous theory has been developed to account for the phenomenon that requires solutions of nonlinear field equations. However, Chakrabarti and Chaudhury have been able to develop a model at a scaling level that captures the main physics underlying the phenomenon.
Chakrabarti anticipates completing her Ph.D. in 2017 and pursuing an academic career. She also published a Langmuir paper with Chaudhury in May 2013 that describes their analysis of elastocapillarity in adhesion-induced instability in thin films and the surface tension of the soft elastic hydrogel that she used in her later experiments.
Since the second Langmuir paper was published, Chakrabarti and Chaudhury have continued experimenting with particles in the gel. They have also submitted for publication an article that presents a more detailed model to account for particle interaction that occurs on the gel’s surface.
Photos by Christa Neu
Posted on:
Friday, April 04, 2014